The northern and southern lights—known scientifically as aurora borealis in the Northern Hemisphere and aurora australis in the Southern Hemisphere—are among Earth’s most mesmerizing natural wonders. When conditions are just right, these ethereal ribbons of color appear in the night skies, typically around the Arctic and Antarctic circles, creating a dazzling display driven by powerful space weather phenomena.
Auroras are the result of intense geomagnetic storms that occur when fluctuations in the solar wind—a continuous stream of charged particles from the sun—disturb Earth’s magnetosphere. These energized particles are funnelled along magnetic field lines toward the poles, where they collide with oxygen and nitrogen atoms in the ionosphere. These collisions transfer energy, exciting the atoms. As they return to their normal state, the atoms release photons—tiny particles of light that, in large numbers, paint the sky with the glowing waves we recognize as auroras.
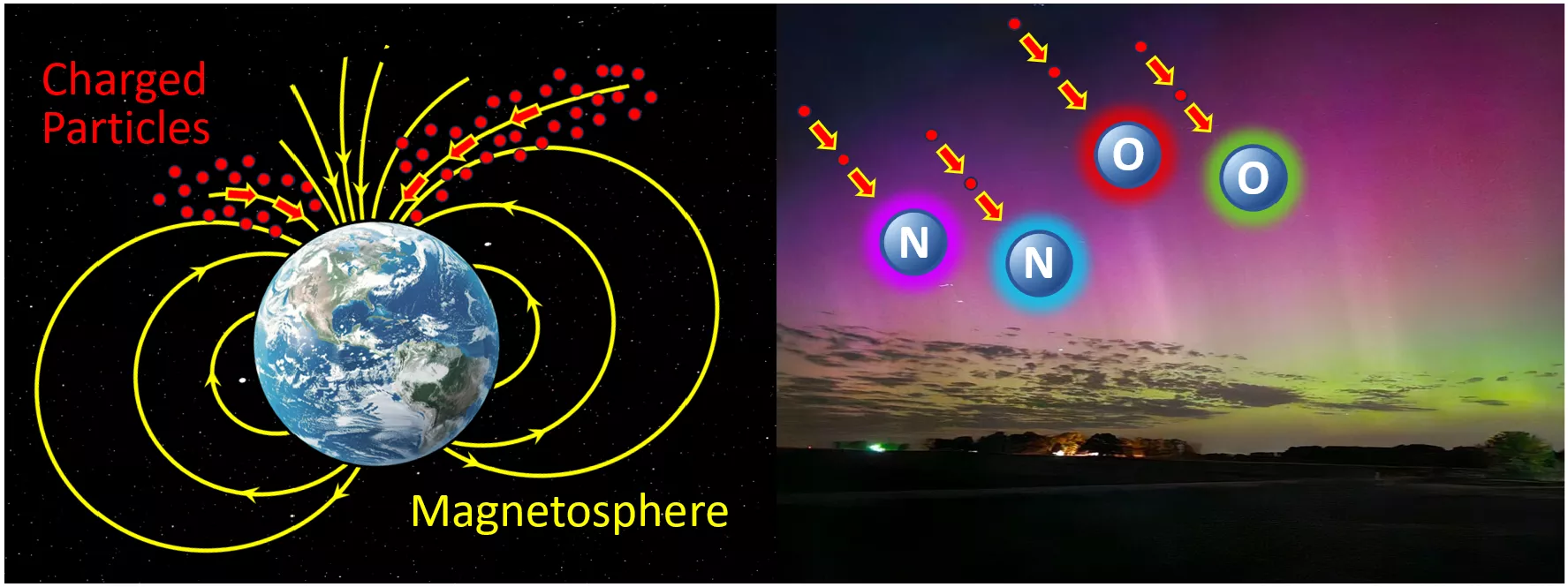
Auroras form when charged particles from the Sun are channeled toward the poles, where they collide with oxygen (O) and nitrogen (N) atoms in our upper atmosphere. These collisions excite the atoms, which then release particles of light energy as they return to their normal state. When billions of such collisions occur, the resulting glow becomes visible to the human eye. The colors produced depend on the type of gas and altitude: oxygen emits green below 150 miles and red above 150 miles, while nitrogen contributes blue hues below 60 miles and purple above 60 miles.
NOAA’s Joint Polar Satellite System (JPSS) Program is comprised of three operational satellites that offer a powerful vantage point for observing auroras. Orbiting Earth from pole to pole in low Earth orbit (LEO), they are ideally positioned to monitor these dynamic light displays. Each satellite—NOAA/NASA Suomi NPP, NOAA-20, and NOAA-21—carries the Visible Infrared Imaging Radiometer Suite (VIIRS) equipped with a unique Day/Night Band (DNB) that is sensitive to very low levels of visible light.
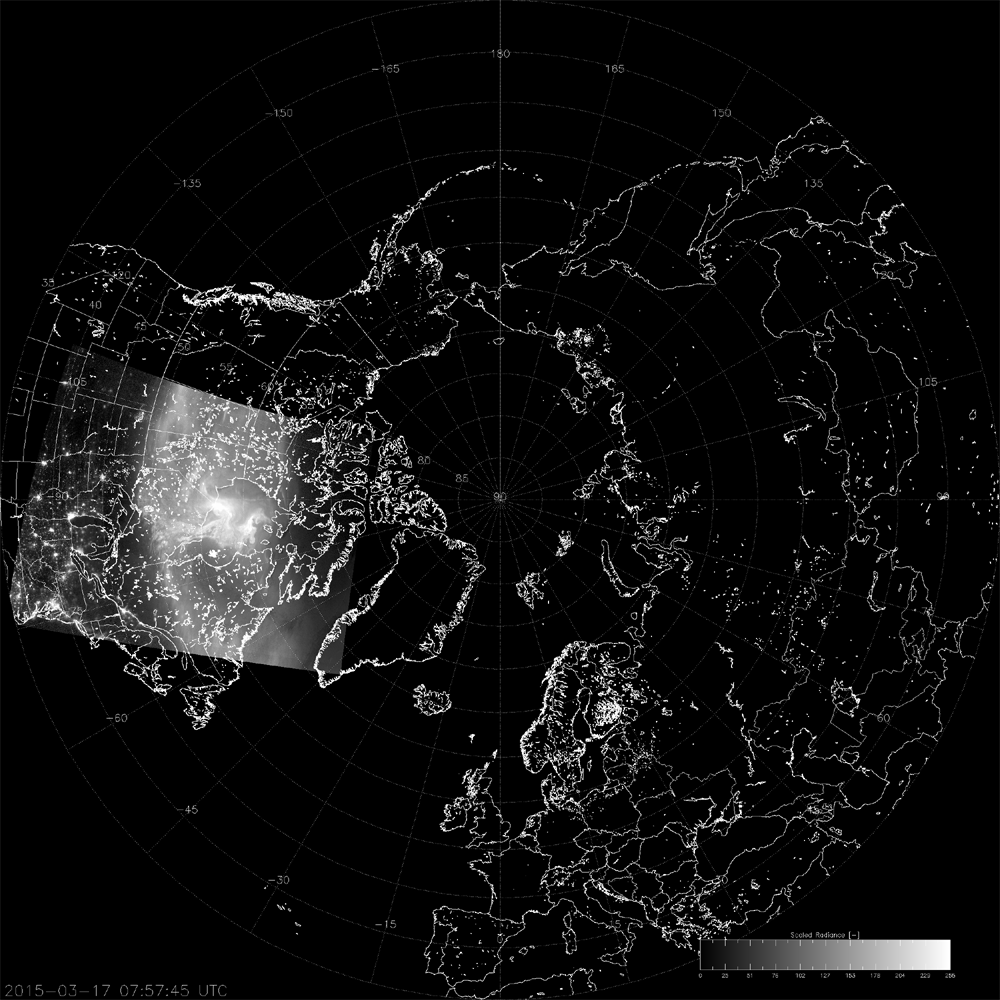
Animation of Suomi NPP VIIRS DNB imagery showing the aurora borealis circling above the North Pole, with the Northern Hemisphere in view and the U.S. on the left. The bright white area is the aurora observed on March 17–18, 2015. Credit: Cooperative Institute for Research in the Atmosphere (CIRA) at Colorado State University (CSU).
The DNB makes it possible to detect auroras from space in remarkable detail, revealing their movement, brightness, shape and spatial extent. In addition to capturing stunning imagery, DNB aurora observations offer valuable insights to help verify aurora forecasts, guide resource planning in aurora tourism regions, and support preparedness for space weather, which can disrupt radio signals, communications, Global Positioning Systems (GPS), power grids and satellite operations.
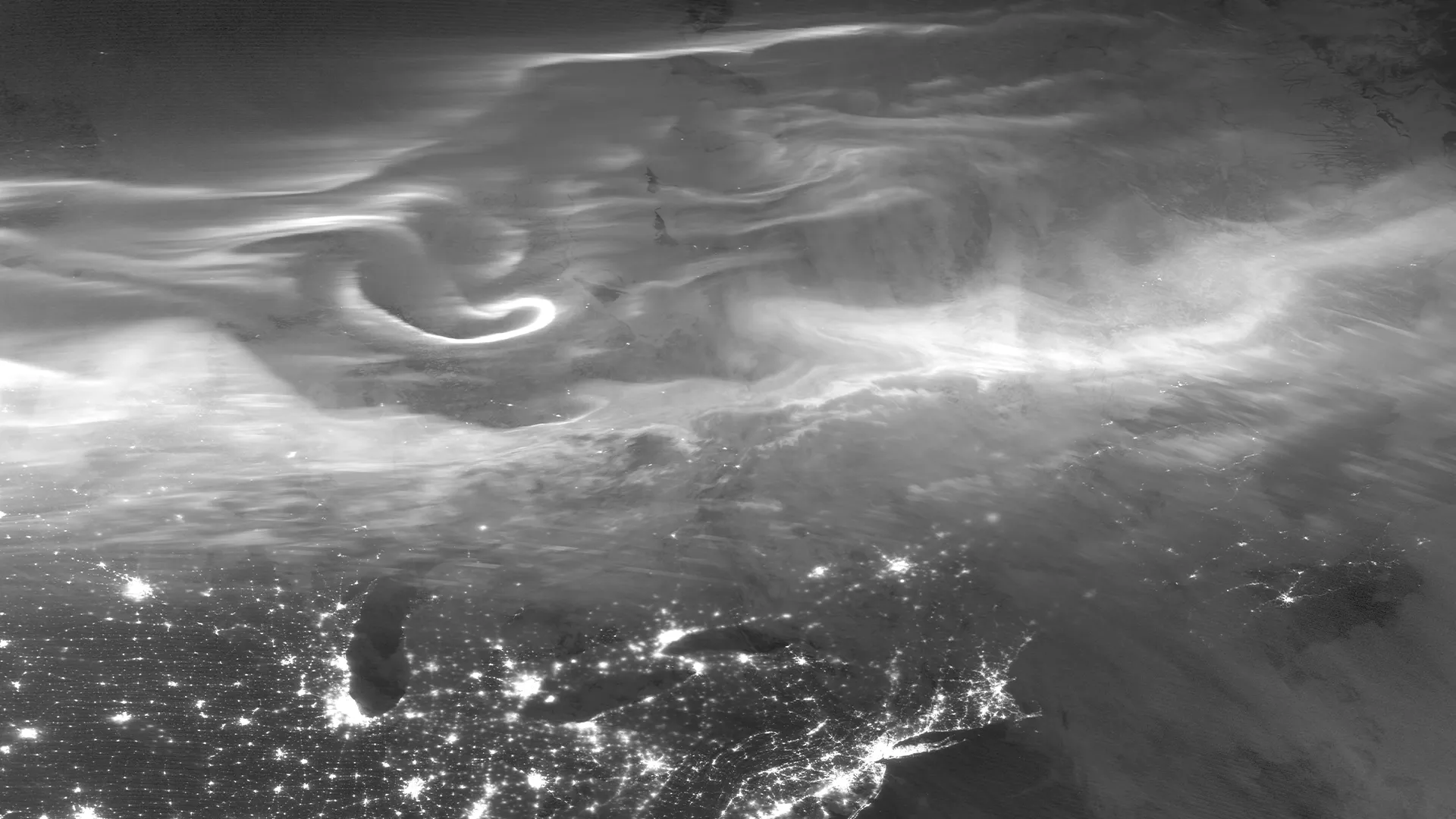
A breathtaking view from NOAA-20 VIIRS DNB shows the aurora borealis over the Eastern U.S. and Canada on April 24, 2023, with city lights glowing beneath the display. Credit: CIRA/CSU.
In 2024, as Solar Cycle 25 reached its peak—known as the solar maximum, the most active phase of the sun’s 11-year cycle—Earth experienced some of the most intense geomagnetic storms in years. The resulting surge in auroral activity earned 2024 the unofficial title of “the year of the aurora,” with brilliant displays occurring more frequently and appearing farther south than where they are normally seen in high-latitudes.
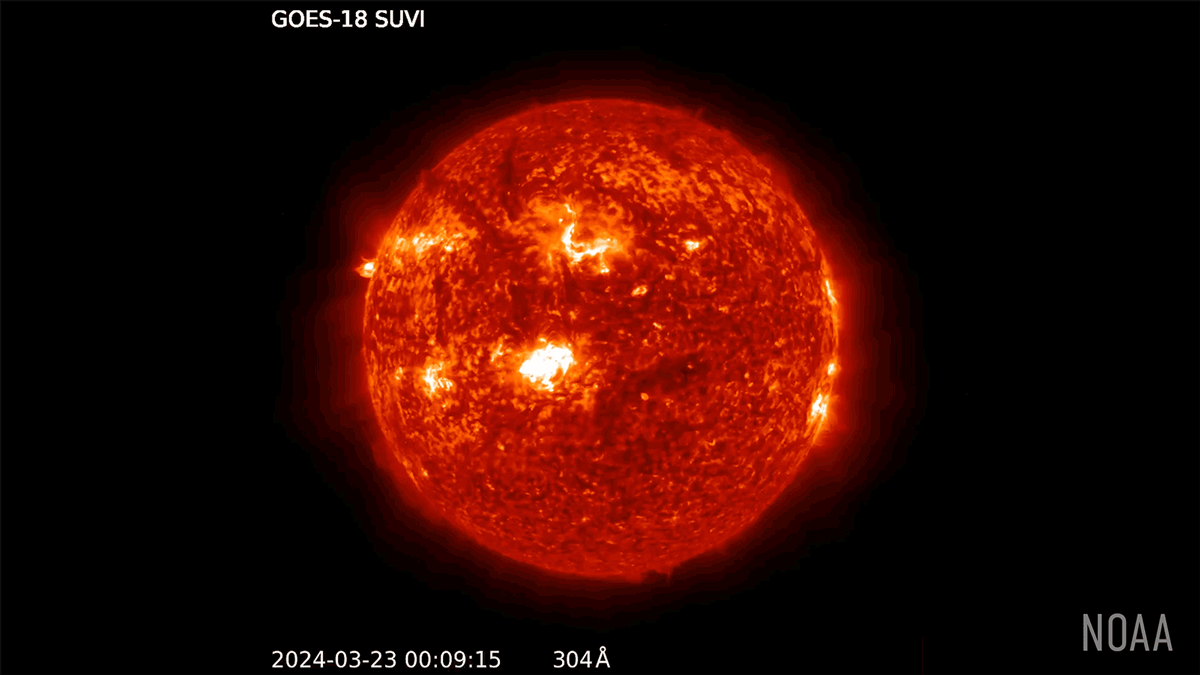
The GOES-18 Solar Ultraviolet Imager (SUVI) observed numerous solar flares from March 23-24, 2024, including a powerful X-class solar flare—the most intense category. A surge of extremely hot plasma, known as a coronal mass ejection (CME), also erupted and raced toward Earth resulting in geomagnetic storms and vivid auroras. Credit: NOAA NESDIS.
Some auroras stretched so far beyond their typical high-latitude boundaries that they were visible in unexpected places, including the Southern U.S. While people watched in awe from the ground, VIIRS captured the full scope of the events from above, contributing to a better understanding of the processes behind this captivating phenomenon.
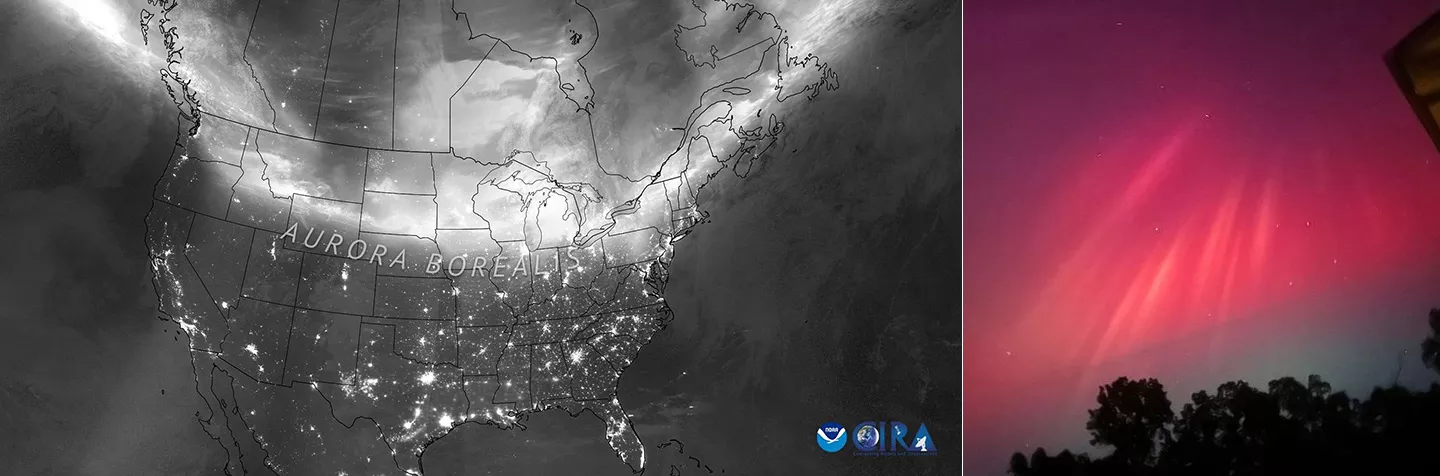
Left: VIIRS DNB imagery captures the aurora borealis stretching over the U.S. and Canada during the night of May 10, 2024, and into the early hours of May 11. Credit: CIRA/CSU. Right: The aurora as seen from Viola, Arkansas on the night of May 10, 2024. Credit: Hunter Belvedresi (Hunalbe19), CC BY 4.0.
Since its debut in 2012, the VIIRS DNB has transformed how we observe and understand nighttime conditions from orbit. In addition to observing auroras, its low-light capabilities are essential for monitoring rapidly evolving hazards that can emerge overnight—such as wildfires, smoke plumes, low clouds and fog, and sea ice—supporting enhanced disaster response and public safety. The DNB also plays a vital role in economic and national security applications by providing data to track power outages, illegal maritime activity, human migration, and even economic activity.
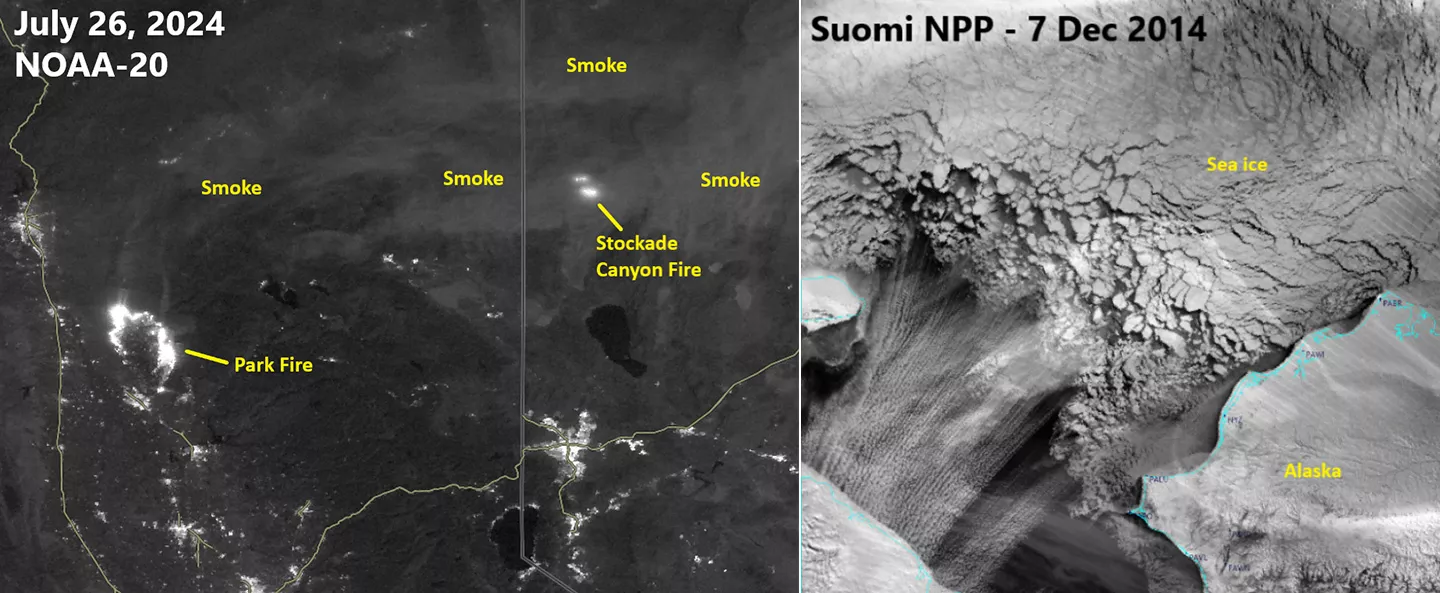
Left: NOAA-20 VIIRS DNB imagery from July 26, 2024, highlights wildfire hotspots and smoke from the Park Fire in Northern California and the Stockdale Canyon Fire in Northwestern Nevada. Source: NASA Worldview. Right: Suomi NPP VIIRS DNB imagery from December 7, 2014, shows a massive amount of drift ice in the Chukchi Sea, off Alaska’s northwest coast. Credit: Cooperative Institute for Meteorological Satellite Studies (CIMSS) at University of Wisconsin.
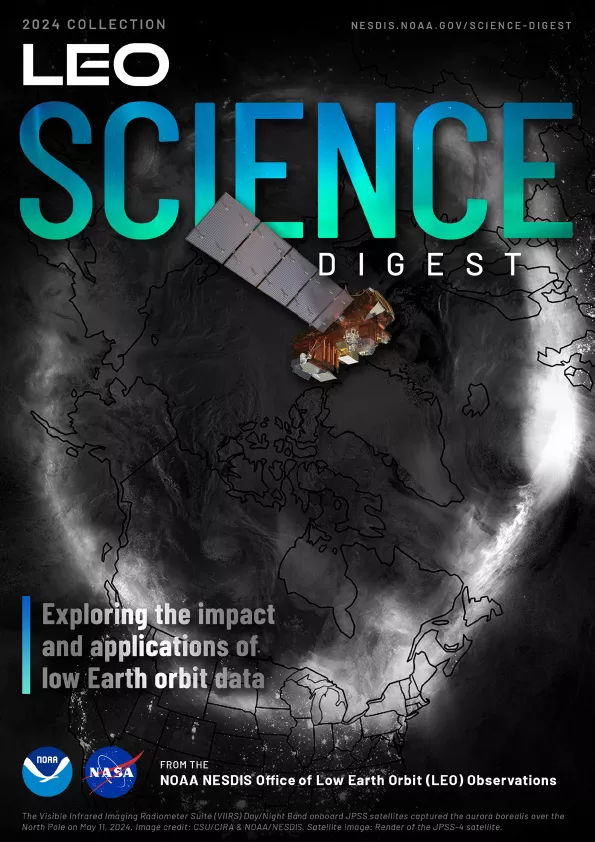
With two additional VIIRS instruments planned for launch in 2027 and 2032, this essential observation capability is set to continue for years to come. As our ability to monitor Earth’s atmosphere, oceans and land at night expands, so too does our understanding of the dynamic processes that shape them. To explore the VIIRS Day/Night Band in more detail—including stunning aurora imagery and insights into solar activity and space weather—read the article Capturing the Magic of Auroras From Space With the VIIRS Day/Night Band in the 2024 LEO Science Digest.